Laser spectroscopy of muonic atoms
Muonic atoms are hydrogen-like atomic bound systems formed by a negative muon and a nucleus.
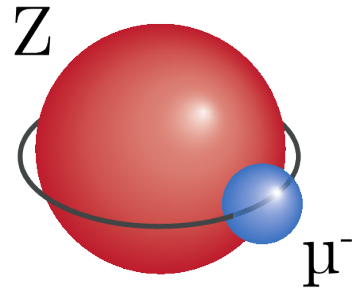
Because the muon mass is 200 times larger than the electron mass, the Bohr radius of the muonic atoms is 200 times smaller than the Bohr radius of the electrons in regular electronic atoms. Because of the considerable overlap between the muonic and the nuclear wave-functions, the energy levels of the muonic atoms show a high sensitivity to the nuclear structure, in particular to the size of the nucleus. Spectroscopy of muonic atoms is thus a powerful tool to determine nuclear charge radii with high precision.
While spectroscopy of medium- and high-Z muonic atoms has been performed by measuring the characteristic X-rays emitted in the de-excitation process of the muonic atoms following formation, we have concentrated our effort in performing laser spectroscopy of light muonic atoms.
Muonic hydrogen and the proton radius puzzle
There are three methods to determine the proton charge radius. The historical approach is via elastic electron-proton scattering. The second approach is high-precision laser spectroscopy in hydrogen while the third approach is based on pulsed laser spectroscopy of muonic hydrogen (an atom formed by a muon and a proton, μp).

The value of the proton radius that we extracted from μp is an order of magnitude more precise than the values obtained from the other methods. Yet, we face a discrepancy of about 6σ as visible in Figure 2. This discrepancy, referred to as the ``proton radius puzzle’’, has prompted various investigations in the context of bound-state QED, the proton structure, atomic spectroscopy, beyond-standard-model physics, scattering experiments and atomic spectroscopy. Our recent measurement of the 2S-2P transition in muonic deuterium combined with the isotopic shift in hydrogen, has confirmed our value of the proton charge radius as extracted directly from μp.
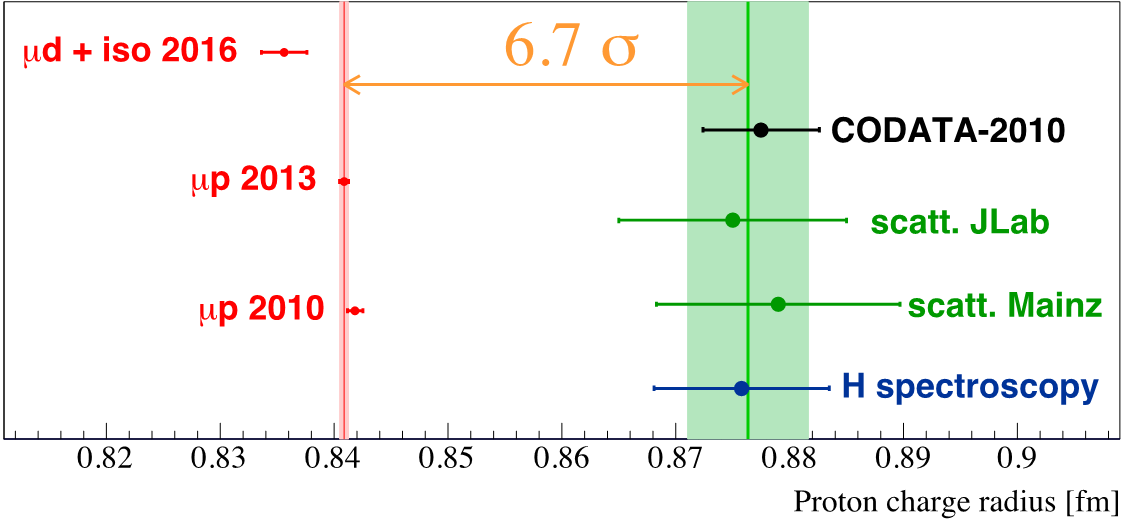
Muonic Helium Spectroscopy
After the successful measurement of the 2S-2P energy splitting in muonic hydrogen and muonic deuterium we have addressed the 2S-2P energy splitting in muonic helium. Successful beam times have been carried out at the piE5 beam line at PSI in 2013 and 2014, within the international CREMA collaboration.
The measurements will allow to extract with high precision the charge radii of the 4He and 3He nuclei, to shed light on the "proton radius puzzle", benchmark few nucleon models of light nuclei and permit precision tests of higher-order bound-state quantum electrodynamics contributions.
Measurement of the Hyperfine Splitting (HFS) in Muonic Hydrogen
The 2S-2P transition measurements in muonic hydrogen (μp) and muonic helium yielded precise values of the proton and helium nucleus charge radii, i.e., provided information on the charge distribution of the proton and helium nucleus.
Differently, the HFS of μp, which arises from the interaction between the magnetic moment of the muon and the magnetic moment of the proton, carries information about the magnetic structure of the proton.
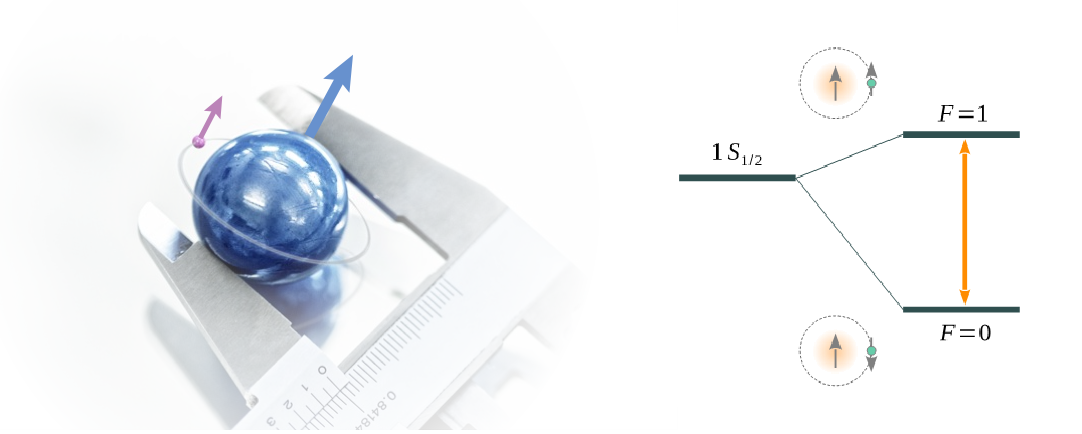
Our goal is to measure the ground state HFS in μp by means of pulsed laser spectroscopy with a relative accuracy of 1x10-6 to deduce the two-photon exchange contribution (sum of Zeemach radius and polarizability contributions) with a relative accuracy of 1x10-4.
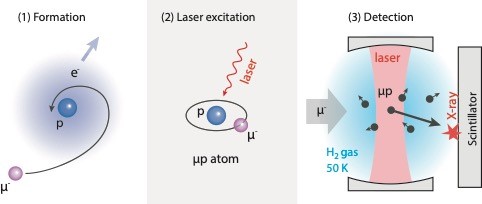
In a nutshell our experiment works as follows (see Figure 4). Low energy muons (μ− ) are stopped in a cryogenic H2 gas target at 1 bar pressure, whereby μp atoms in highly excited states are formed. The highly excited μp atoms quickly de-excite to the F=0 (singlet state) of the ground state. A laser pulse induces the hyperfine transition from the lower lying singlet state F = 0 into the higher lying triplet state F=1 i.e.,
µp(F=0) + ɣ → µp(F=1) .
Shortly afterwards, the higher lying triplet state (F=1) is collisionally quenched back to the singlet state. In this process, the HFS transition energy is converted into kinetic energy.
µp(F=1) + H2→µp(F=0) + H2+ Ekin .
For a sufficiently small target, the faster moving laser-excited μp atoms reach the target walls coated with high-Z material. At the walls, the muon transfers from μp to a high-Z atom leading to the formation of a highly excited muonic high-Z atom (μZ)∗ which promptly de-excites emitting X-rays. A resonance curve is retrieved by plotting the number of emitted X-rays as a function of the laser frequency.
The experimental setup we are developing for this measurement consists of three main components:
• A laser system delivering pulses of 5 mJ at a wavelength of 6.8 μm and a bandwidth smaller than 100 MHz with a delay between trigger and emission of the pulse shorter than 1μs. This laser system rests upon two cutting-edge technologies: thin-disk lasers (TDL) and parametric down-conversion stages
• A multi-pass optical cavity enhancing the laser fluence and operating at cryogenic temperatures.
• A detection system that measures the muonic X-rays with high efficiency while supressing the background caused by electron from muon decay.
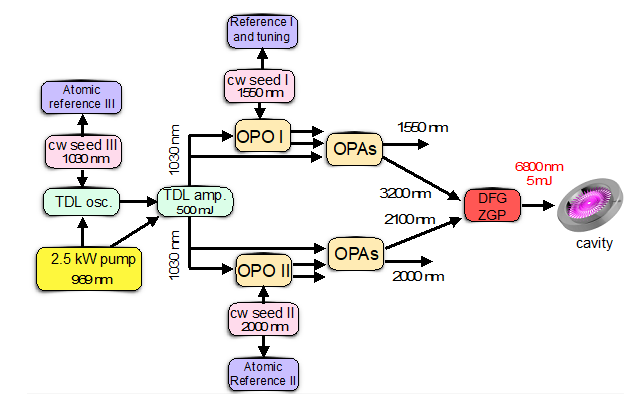
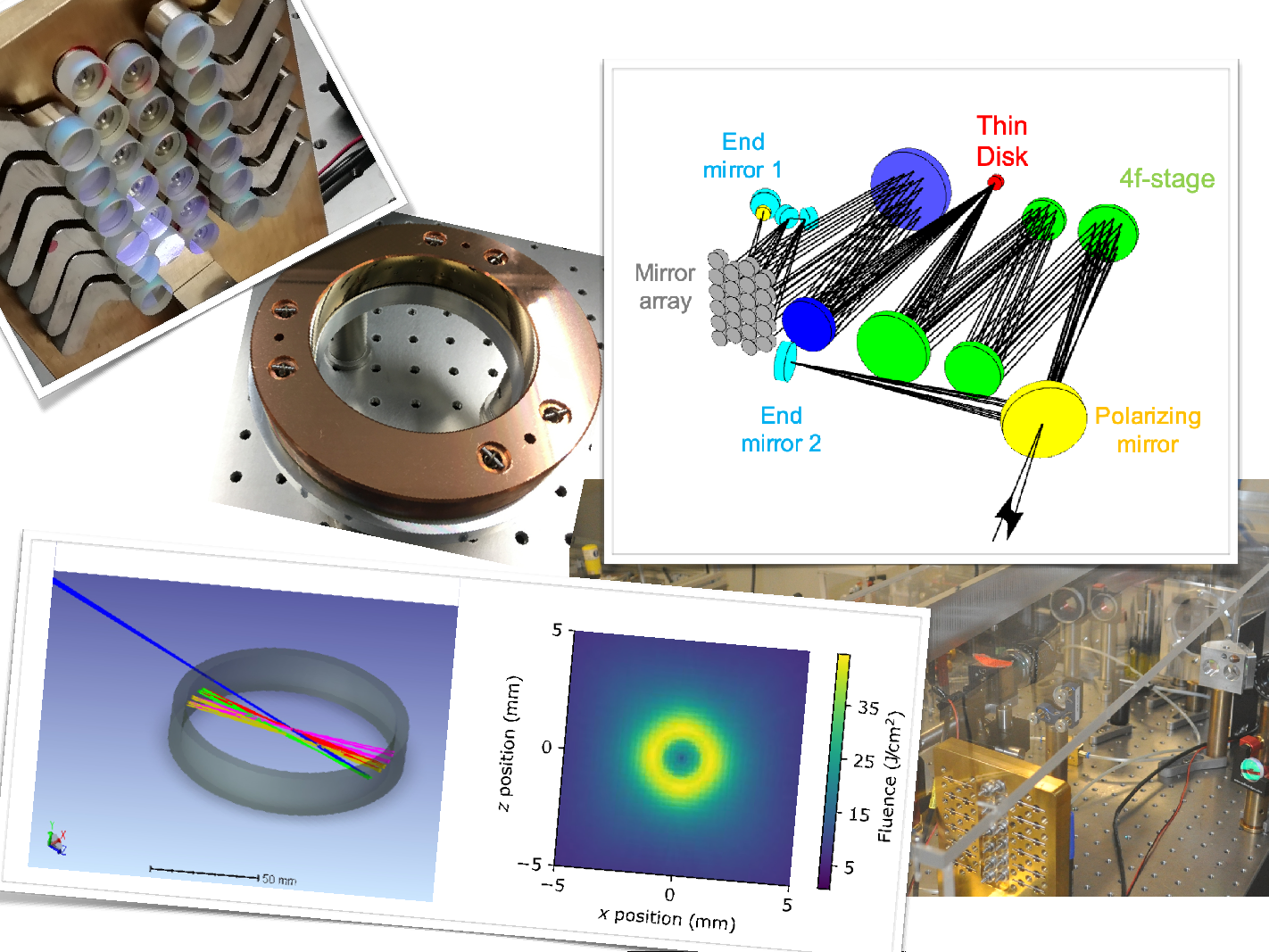